Enzymes
Proteins make up almost all enzymes. Some nucleic acids have enzyme-like properties. Ribozymes are the name for these proteins. A line diagram can be used to represent an enzyme. An enzyme, like any other protein, has a primary structure, which is the amino acid sequence. Secondary and tertiary structures are present in enzymes, as they are in other proteins. When you look at a tertiary structure, you'll observe that the protein chain's backbone folds in on itself, the chain crisscrosses itself, and many crevices or pockets form. The 'active site' is one such pocket. An enzyme's active site is a crevice or pocket that the substrate fits into. As a result, enzymes catalyze processes at a rapid pace through their active site.
In many aspects, enzyme catalysts differ from inorganic catalysts, but one key distinction stands out. At high temperatures and pressures, inorganic catalysts perform efficiently, but enzymes are destroyed at high temperatures (say, above 40°C). Enzymes extracted from organisms that dwell in severe heat (e.g., hot vents and sulphur springs) are stable and keep their catalytic power even at extreme temperatures (up to 80°-90°C). The capacity of such enzymes extracted from thermophilic organisms to maintain their thermal stability is thus critical.
Chemical reactions
Chemical compounds go through two sorts of transformations. A physical change is merely a change in shape that does not involve the breaking of connections.This is a bodily function. A change in state of matter is another physical process, such as when ice melts into water or when water becomes vapour. These are physical processes as well. A chemical reaction, on the other hand, occurs when bonds are broken and new bonds are generated during transformation. For example
Ba (OH)2+ H2SO4 ® BaSO4 + 2H2O
is a chemical process that takes place in an inorganic state. The conversion of starch to glucose is also an organic chemical reaction. The amount of product created per unit time is referred to as the rate of a physical or chemical process. It can be written as:
rate=dP/ dT
If the direction is indicated, rate is also known as velocity. Temperature, among other things, affects the rates of physical and chemical processes. For every 10°C change in either direction, the rate doubles or declines by half, according to a common rule of thumb. Catalyzed reactions proceed at a much faster rate than uncatalyzed reactions. For every 10°C change in either direction, the rate of enzyme catalysed reactions would be substantially larger than the same but uncatalyzed reaction. Catalyzed reactions proceed at a much faster rate than uncatalyzed reactions. When enzyme-catalyzed reactions are detected, the rate is much higher than when the identical process is not catalysed. As an example,
In the absence of any enzyme, this reaction is extremely sluggish, producing just around 200 molecules of H2CO3 per hour. The reaction speeds up substantially when carbonic anhydrase, an enzyme found in the cytoplasm, is used, with roughly 600,000 molecules generated every second. The enzyme has sped up the reaction rate by a factor of ten million. The power of enzymes is very amazing! Thousands of different enzymes exist, each catalysing a different chemical or metabolic reaction. A metabolic route is a multistep chemical reaction in which each step is catalysed by the same enzyme complex or separate enzymes. As an example,
is a metabolic pathway in which glucose is converted to pyruvic acid via 10 enzyme-catalyzed chemical events. At this point, you should be aware that this metabolic pathway, when supplemented with one or two additional processes, produces a wide range of metabolic end products. Lactic acid is produced in skeletal muscle under anaerobic circumstances. Pyruvic acid is generated under typical aerobic circumstances. The similar route leads to the generation of ethanol in yeast during fermentation (alcohol). As a result, different products are possible depending on the circumstances.
How do Enzymes bring about such High Rates of Chemical Conversions?
The concept of an active site is already known. A reaction is referred to as a chemical or metabolic change. A'substrate' is the chemical that is transformed into a product. As a result, enzymes, which are three-dimensional proteins with a 'active site,' convert a substrate (S) into a product (P). This can be represented symbolically as
Within a specific cleft or pocket, the substrate 'S' must bind the enzyme at its'active site.' The substrate must diffuse into the 'active site.' As a result, the creation of a 'ES' complex is required. The letter E stands for enzyme. This intricate structure is a one-time occurrence. A new structure of the substrate called the transition state structure is created when the substrate is attached to the enzyme active site. The product is quickly removed from the active site after the intended bond breaking/making is completed. In other words, the substrate's structure is changed into the product's structure (s). This transformation must pass via a structure known as a transition state structure.Between the stable substrate and the result, there could be many additional 'altered structural states.' The notion that all other intermediate structural states are unstable is implicit in this assertion. Stability has to do with the molecule's or structure's energy status.

The potential energy content is shown by the y-axis. The x-axis depicts the structural transformation or states as they proceed through the 'transition stage.' There would be two things that you would notice. The difference in energy levels between S and P. When 'P' is less than 'S,' the reaction is exothermic. It is not necessary to provide energy (through heating) in order to manufacture the product. Regardless of whether the reaction is exothermic or spontaneous or endothermic or energy-demanding, the 'S' must pass through a significantly higher energy level or transition state. 'Activation energy' is the difference between the average energy content of 'S' and that of this transition state. Enzymes gradually break down this energy barrier, allowing the 'S' to become 'P' more easily.
Nature of Enzyme Action
In order to create a highly reactive enzyme-substrate complex (ES), each enzyme (E) has a substrate (S) binding site in its molecule. This complex has a brief half-life and dissociates into its product(s) P and the unmodified enzyme, with the enzyme-product complex forming in the middle (EP). Catalysis requires the creation of the ES complex.
E+S → ES → EP → E+P
An enzyme's catalytic cycle can be broken down into the following steps:
1. The substrate first binds to the enzyme's active site, fitting into the active site.
2. The enzyme's shape changes as a result of the substrate's binding, causing it to fit more tightly around the substrate.
3. The enzyme's active site, which is now in close proximity to the substrate, breaks the substrate's chemical bonds, forming a new enzyme-product complex.
4. The reaction products are released by the enzyme, and the free enzyme is ready to bind to another molecule of the substrate and repeat the catalytic cycle.
Factors Affecting Enzyme Activity
A change in the circumstances can impact the activity of an enzyme by altering the protein's tertiary structure. Temperature, pH, changes in substrate concentration, and the binding of certain molecules that affect its activity are examples of these.
Temperature and pH: Enzymes typically operate within a narrow temperature and pH range. The optimum temperature and pH for each enzyme are defined as the temperature and pH at which the enzyme is most active. Both below and above the ideal value, activity decreases. Because proteins are denatured by heat, low temperatures maintain the enzyme in a temporarily inactive state, but high temperatures eliminate enzymatic function.

Substrate Concentration: At first, the enzymatic reaction's velocity increases as the substrate concentration rises. The reaction eventually achieves a maximum velocity (Vmax) that isn't exceeded by any further increase in substrate concentration. This is because there are fewer enzyme molecules than substrate molecules, and there are no free enzyme molecules to interact with the additional substrate molecules after these molecules have been saturated. An enzyme's activity is also affected by the presence of certain molecules that bind to it. The process of inhibition occurs when a chemical binds to an enzyme, and the chemical is referred to as an inhibitor.Competitive inhibitors are those that have a chemical structure that closely mimics that of the substrate and inhibits the enzyme's activity. Because of its structural similarity to the substrate, the inhibitor competes with it for the enzyme's substrate binding site. As a result, the substrate is unable to attach, and the enzyme's activity is reduced, as seen in the inhibition of succinic dehydrogenase by malonate, which is structurally similar to the substrate succinate. Competitive inhibitors like these are frequently employed to combat bacterial infections.
Classification and Nomenclature of Enzymes
There have been thousands of enzymes identified, isolated, and analyzed. The majority of these enzymes have been divided into groups depending on the kind of reactions that they catalyze. Enzymes are classified into six classes, each having four to thirteen subclasses, and given a four-digit number.
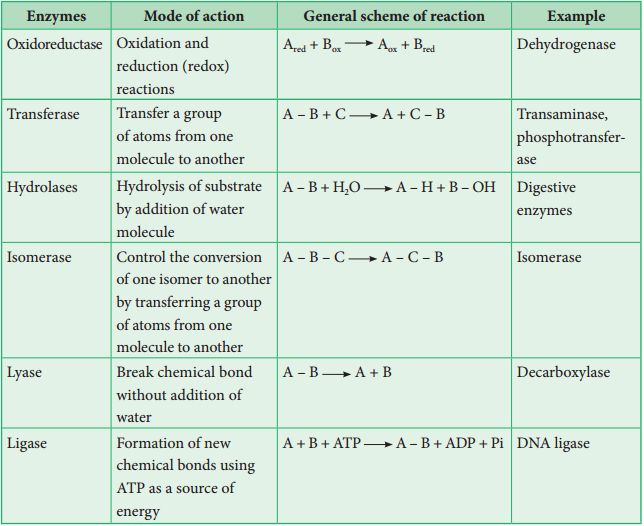
Co-factors
Enzymes are polypeptide chains made up of one or more polypeptide chains. However, in other situations, non-protein elements known as cofactors are coupled to the enzyme in order for it to be catalytically active. The apoenzyme refers to the protein part of the enzyme in these cases. Prosthetic groups, co-enzymes, and metal ions are all examples of cofactors. Prosthetic groups are chemical molecules that are strongly attached to the apoenzyme, distinguishing them from other cofactors. The prosthetic group haem is a component of the active site of enzymes like peroxidase and catalase, which catalyze the breakdown of hydrogen peroxide to water and oxygen.Co-enzymes are organic substances as well, although their relationship with the apoenzyme is very temporary, occurring most often during catalysis. Co-enzymes are also used as co-factors in a variety of enzyme-catalyzed processes. Many coenzymes contain vitamins as important chemical components; for example, the coenzymes nicotinamide adenine dinucleotide (NAD) and NADP contain the vitamin niacin. Metal ions that create coordination bonds with side chains in the active site while also forming one or more coordination bonds with the substrate are required for the activity of a number of enzymes, for example, zinc is a cofactor for the proteolytic enzyme carboxypeptidase. When a co-factor is removed from an enzyme, its catalytic activity is lost, indicating that they play an important part in the enzyme's catalytic activity.